Introduction
Consumers prefer meat products since they are rich in protein, lipids, vitamins, minerals, and bioactive hydrolysates—all of which are necessary for a balanced and healthful diet. Apart from serving as a primary energy source, fats also significantly influence the sensory attributes and texture of the final product (Serdaroğlu, 2006). Nevertheless, the elevated intake of saturated fats from meat consumption has been linked to cardiovascular diseases, obesity, and other chronic health conditions (Chomanov et al., 2022). The World Health Organization advises restricting daily energy intake from fats to 15%−30%, wherein saturated fat consumption should not surpass 10%, with the remaining portion being composed of mono and polyunsaturated fatty acids (PUFA; WHO, 2018). As awareness of the role of diet as a key determinant of lifespan increases, there is a growing number of conscious consumers following WHO guidelines (WHO, 2013). As a result, many studies within the meat industry concentrate on diminishing fat content and/or enhancing fatty acid profiles. One approach involves partially replacing animal fats with vegetable oils, reducing saturated fatty acid levels, and increasing PUFAs (Guo et al., 2023). However, direct enrichment with vegetable oils has drawbacks leading to organoleptic and technological issues, affecting the texture of the final meat product (Domínguez et al., 2016).
Recently oleogel has been considered as a technique showing the most promise for structuring oil as a method of incorporating vegetable oils into meat systems (López-Pedrouso et al., 2021) Oleogels exhibit a three-dimensional trapping capacity for liquid oil at very low concentrations (1%–10%) (Thakur et al., 2022). Oleogels, derived by using various structurants from different plant oils (such as sunflower, corn oil, etc.), are utilized to achieve the desired textural (especially hardness) and sensory properties (Guo et al., 2023; Xu et al., 2022). Simultaneously, oleogels offer an opportunity to enhance the fatty acid profile using healthy oils in the formulation (Gómez-Estaca et al., 2019; Morales et al., 2023). The research regarding the influence of oleogels on different characteristics of meat products is progressing, with a significant focus on their substitution for animal fat in bologna sausages (da Silva et al., 2019), paté (Martins et al., 2020), Frankfurter-type sausages (Wolfer et al., 2018; Zetzl et al., 2012), burgers (Adili et al., 2020; Khiabani et al., 2020), and meatballs (Oh et al., 2019).
The pomegranate seed oil contains valuable punicic acid, alongside other unsaturated fatty acids, phytosterols, and tocopherols. It is recognized for its antioxidant, antimicrobial, immunomodulatory, anticancer, and lipid metabolism-regulating properties (Boroushaki et al., 2016). Pomegranate seed oil has been utilized in animal nutrition (Banaszkiewicz et al., 2018; Szymczyk and Szczurek, 2016), food packaging (Morais et al., 2020; Sogut et al., 2019), and functional components in food formulations (Esther Lydia et al., 2020; Mohagheghi et al., 2011; Siraj et al., 2019), acting as an antimicrobial agent (Amri et al., 2020; Lu et al., 2020) and a substitute for fat in chocolate formulations (Fayaz et al., 2017a, Fayaz et al., 2017b). Although pomegranate seed oil has been used in sausage formulation (Hoseini et al., 2020), there is no study found where oleogel formulated with pomegranate seed oil and chitosan has been used in meat products.
Chitosan, a copolymer obtained through partial or complete deacetylation of chitin, is easily found in shellfish, exhibiting superior properties compared to many other biopolymers due to its availability, non-toxic nature, microorganism inhibition, biodegradability, biocompatibility, and unique chemical and physical characteristics (Ke et al., 2021). Additionally, chitosan has been reported to possess broad-spectrum activities, such as antibacterial, antifungal, and antiviral properties (Ke et al., 2021; Özdemir, 2014). Chitosan’s increasing popularity across various applications (stabilizer, gelling agent, binder, dispersing agent, thickener, lubricant, drug carrier, etc.) is attributed to its versatility (Özdemir, 2014). While there is growing interest in chitosan, and it has been used in various areas, including stabilizers, gelling agents, binders, dispersing agents, thickeners, lubricants, and drug carriers, no study has been found where chitosan is utilized as an oleogelator.
In light of this information, this study aims to investigate the effects of using oleogel formulated with chitosan and pomegranate seed oil in model meat systems as a replacer for animal fat on chemical composition, technological and textural properties, as well as lipid and protein oxidation.
Materials and Methods
Beef (73.6% moisture, 20.7% protein, 4.2% fat, and 1.5% ash) and beef fat (95.7% lipid, 4.2% moisture, and 0.1% ash) were purchased from a local butcher in Izmir to produce the model system meat emulsions (MEs). To produce oleogel, chitosan (deacetylation degree 80%) and pomegranate seed oil (palmitic acid (8.0%), stearic acid (3.87%), oleic acid (14.0%), linoleic acid (15.22%), punicic acid (50.17%) were supplied from Nurbal Şifa Aktar Natural Food Industry Trade (Istanbul, Turkey) and Smart Kimya Tic. ve Dan. (Izmir, Turkey), respectively. Curing agents were purchased from Fansada Aroma and Spice Food Products (Ankara, Turkey). Analytical-grade chemicals sourced from Sigma-Aldrich Chemie GmbH (Schnelldorf, Germany) were utilized in the experiments.
The oleogel (Fig. 1) was prepared using the components of chitosan: pomegranate seed oil: water in a ratio of 2:5:5, referencing the study conducted by da Silva et al. (2019). Firstly, chitosan and water were mixed with a magnetic stirrer (MSH-20A, Witeg Labortechnik GmbH, Wertheim, Germany), for 6 min. Then, this mixture was heated for 15 min at 75°C in a water-bath (Nüve, Ankara, Turkey). After the heating process, pomegranate seed oil was added dropwise, and a blender (Sinbo, Istanbul, Turkey) was used to homogenize the mixture for 5 min. Finally, the homogeneous mixture was allowed to cool overnight at +4°C.
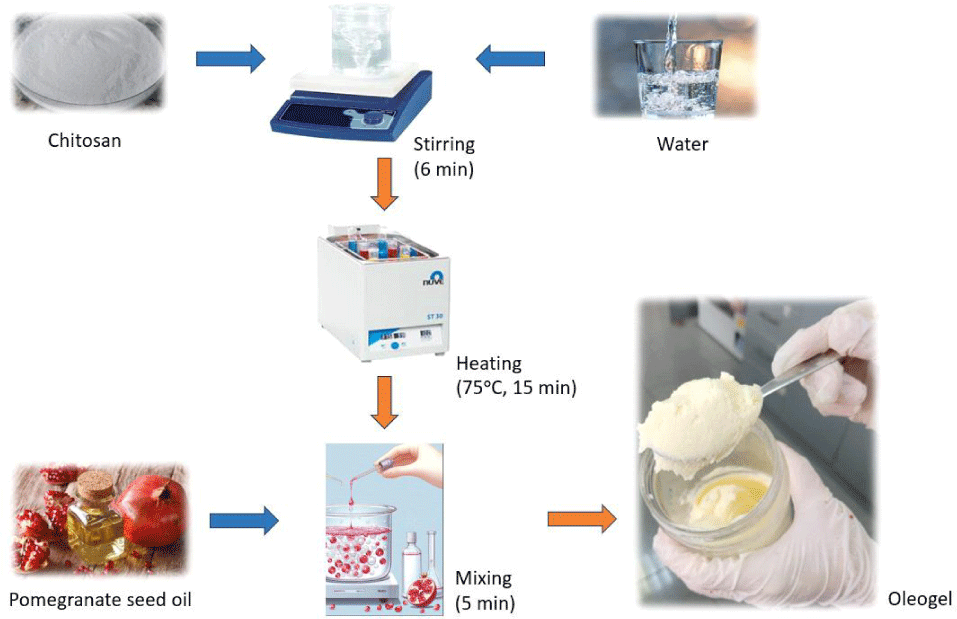
Model system MEs were produced according to Zungur et al. (2015), using beef fat and/or oleogel as a fat replacer. The treatment combinations comprised four distinct formulations, outlined as follows: (1) ME formulated with 100% beef fat (control-C), (2) ME formulated with 50% oleogel (OG50), (3) ME prepared with 75% oleogel (OG75) and (4) ME prepared with 100% oleogel (OG100). Four treatments were produced twice on separate days according to Table 1. The beef and beef fat were ground through a 3 mm grinder plate (Arnica W2000 Grande, Istanbul, Turkey). Then the minced beef was homogenized with a Thermomix (Thermomix, Vorwerk, Germany) at 39×g for a min. After that, NaCl, STTP, and sodium nitrite were added and homogenized at 39×g for 2 min. Afterward, half of the ice, beef fat, and/or oleogel were added and stirred at 188×g for 3 min. After that, the rest of the ice was added, and the process was carried out for 3 more min. Then, the meat batter was emulsified at 622×g for a min. To eliminate any air bubbles, MEs were put in centrifuge tubes (50 mL) and then centrifuged at 971×g for a min (Nüve, NF 400). After that, the MEs were heated at 70°C in a water-bath (Nüve) for 30 min. Finally, the MEs were allowed to cool down to room temperature.
The rotational viscometer was employed to measure the dynamic viscosity of the oleogel. To analyze the size distribution of oil globules, a Malvern Mastersizer 2000S equipped with a He-Ne laser (with a wavelength of 623 nm) was utilized. Image capture was facilitated by an Olympus SLR-E330 digital color camera paired with a light microscope (Olympus CX21, Tokyo, Japan) featuring a 100× lens.
To determine the thermal processing stability, oleogels were incubated in a water bath at 70°C for 30 min, and the resulting phase separation was observed (Surh et al., 2007).
Syneresis was quantified following the methodology outlined by Serdaroglu et al. (2017). The percentage of syneresis (%Syneresis) was calculated using the prescribed formula.
W1: The weight of the half−filled tub
W3: The weight of the tub after wiped with paper
T: The weight of empty tub
Moisture and ash content were specified following the AOAC (2012) method, fat content was assessed using the method outlined by Flynn and Bramblet (1975), and protein content was analyzed through the Dumas burning method utilizing the LECO Protein/Nitrogen Analyzer (model FP-528, Leco, St. Joseph, MI, USA). pH measurements were conducted according to Nacak et al. (2021). The energy value (in kcal) was computed using Atwater values associated with fat (9 kcal/g), carbohydrates (3.87 kcal/g), and protein (4.02 kcal/g) as outlined by Mansour and Khalil in 2000.
Emulsion stability was determined following the protocol outlined by Hughes et al. (1997). The volumes of total expressible fluid (TEF) and fat (EFAT) were determined using the following formula:
Bloukas and Honikel (1992) method was used to measure the jelly and fat separation (JFS) of MEs. 200 g of the emulsion were transferred into glass jars, filtered through a sieve, and subjected to heating using a boiling water bath apparatus (Nüve) until the temperature inside reached 90°C. After being cooled to room temperature, the jars were kept at +4°C for 24 h. Then, the jars were heated again at 45°C for 1 h. The volume was measured after draining the liquid jelly and fat into a volumetric cylinder. The separation of jelly and fat was then calculated as a percentage of the batter’s initial weight.
The lipid phase was isolated from the specimens using the extraction procedure detailed by Flynn and Bramblet (1975). The fatty acid methyl esters were then subjected to analysis via gas chromatography (GC 2010 Plus, Shimadzu, Kyoto, Japan), employing a silica capillary column (SUPELCO SP TM-2560; 0.20 μm/m film thickness, 100 m×0.25 mm i.d.). Initially, helium injector and flame ionization detector was maintained at a temperature of 140°C. Subsequently, the oven temperature was incrementally raised from 140°C to 250°C at a rate of 4°C/min, followed by a 10-min stabilization period at 240°C.
The cholesterol content of the samples was determined according to Yüncü et al. (2022). And the following formula was used to specify the cholesterol content (mg/100 g) (Min et al., 2016):
A1: The absorbance value of the blank
A2: The absorbance value of sample solution
Texture profile analyses were conducted using a TA-XT2 texture analyzer (Stable Micro Systems, Haslemere, UK), where various parameters including hardness (N), springiness (mm), cohesiveness, gumminess (N), and chewiness (N×mm) were measured. The samples, which were cylinders measuring 2.5 cm in height and 2.2 cm in diameter, underwent compression twice to 50% of their original height. This compression was achieved with a post-test speed of 2 mm/s, a crosshead speed of 1 mm/s, and a test speed of 1 mm/s, utilizing a 30 kg load cell.
The microstructure analysis of MEs was conducted utilizing scanning electron microscopy (Thermo Scientific Apreo 2, Waltham, MA, USA). The MEs underwent a sequential process involving drying, grinding into powder, and subsequent placement on a conductive carrier. To enhance conductivity, a gold coating was applied using a surface coating device (QUORUM Q150 RES, Rotary Pumped Coater, East Sussex, UK). Subsequently, the prepared samples were introduced into the SEM unit and subjected to a vacuum. Upon reaching the specified vacuum level (1×10+3 mBar), adjustments were made according to the predetermined voltage, and the device was elevated to a high voltage. The electron beam’s interaction with the sample led to the creation of micrographs.
Color parameters, including CIE luminosity (CIE L*), redness (CIE a*), and yellowness (CIE b*), were assessed using a handheld Konica-Minolta colorimeter (CR-200, Konica Minolta, Tyoko, Japan). The measurements were conducted under a D65 illuminant with a 100-standard observer, with readings taken at four distinct locations across the surface of the sample slices.
The method developed by Witte et al. (1970) was used to measure the TBAR value. 20 g of the sample was homogenized with 20% cold trichloroacetic acid (TCA) solution for 2 min. After adding 50 mL of distilled water, homogenize for an additional min. Then, the slurry was transferred into a 100 mL flask by filtering it through Whatman No. 1 filter paper. Complete the volume to 100 mL with a 1:1 TCA: distilled water ratio. After that, 5 mL of the filtrate and 5 mL of freshly chilled TBA (0.02 M in distilled water) were pipetted into a test tube. After 35 min of 80°C incubation, the tubes were cooled to room temperature. A spectrophotometer (T-60, PG Instruments, Leicestershire, UK) was used to measure the absorbance of the solution at 532 nm in comparison to a blind solution made with a 1:1 TCA-distilled water ratio. The absorbance was multiplied by 5.2 to obtain the TBAR values, which were stated as mg malonaldehyde/kg sample. Every sample was examined three times during each storage period.
The total carbonyl content of the samples was determined following the method by Oliver et al. (1987). 100 mL of 0.15 M KCl were used to homogenize a 10 g of sample and 25 μL of the homogenate was added to each of the two tubes (X and Y). To find the pellet protein concentration, 1 mL of 2 N HCl was combined in the X tube, and 1 mL of DNPH (2,4-Dinitrophenylhydrazine) was added in the Y tube. The samples were incubated for an h, with a 15 min period of intermittent shaking. A milliliter of TCA was then added to precipitate the proteins. Afterward, the samples were centrifuged at 3,885×g for 10 min. The pellets were initially air-dried in a low-temperature oven and then dissolved in 1 mL of 6M guanidine HCl after being rinsed three times with 2 mL (1:1) ethanol: ethyl acetate (3,885×g, 5 min; 15,540×g, 5 min×2). The supernatants were discarded after this. The protein concentration in the X tube was measured at 280 nm with a standard substance of bovine serum albumin. Using an HCl blank solution, the carbonyl content in the Y tube was measured at 370 nm. The samples’ carbonyl content was reported as nm carbonyl/mg protein.
A modification of Ellman (1959) method was used to specify the amount of sulfhydryl (thiol) in the samples. 0.5 g of the sample was homogenized using 10 mL of 0.05 M phosphate buffer (pH 7.2) following this. 1 mL of the homogenate was taken and combined with 9 mL of phosphate buffer that contained 6 mM ethylenediaminetetraacetic acid, 0.6 M NaCl, and 8 M urea. In a chilled centrifuge, the mixture was centrifuged at 30,459×g for 15 min. After treating a 3 mL aliquot of the supernatant with 0.01 M DTNB (5,5’-dithiobis 2-nitrobenzoic acid), which was made with sodium acetate (0.04 mL), the mixture was incubated at 40°C for 15 min. After the sample was incubated, its absorbance at a wavelength of 412 nm was measured.
The data of the study was evaluated using the General Linear Model procedure within the SPSS software (version 22.0, IBM, Armonk, NY, USA). Four distinct treatments (C, OG50, OG75, and OG100) and various storage durations (0, 3, 6, 9, and 12 days) were designated as fixed effects for each replication, encompassing two separate production batches. Quality parameter analyses were carried out in triplicate for each independent batch. To assess the influence of fat reduction and/or the application of oleogel on quality attributes, one-way analysis of variances (ANOVA) was conducted. Furthermore, two-way ANOVA (ANOVA, GraphPad Software, San Diego, CA, USA) was applied to explore the impacts of treatments and storage conditions. Formulation groups and storage duration (specifically for color and oxidation analysis) were defined as fixed factors, while replications were accounted for as random effects. The significance of a fixed factor prompted the comparison of means using Duncan’s Multiple Test at a 95% confidence level.
Results and Discussion
Characteristics of oleogel are given in Table 2. The pH value of the oleogel was determined as 6.25. In a study, the pH value of the oleogel containing pork skin and high oleic sunflower oil was recorded as 5.80 (da Silva et al., 2019). In another study, oleogel produced using corn oil, sodium caseinate, and flaxseed gum was recorded with a pH value of 6.84 (Elbir, 2021). The variation in pH values is believed to stem from the differences in the components used in the oleogel formulation. The determination of color values for petroleum jelly is important due to its potential to influence the color of the product. The CIE L*, CIE a*, and CIE b* values of oleogel were determined as 78.81±0.10, –2.93±0.02, and 18.01±0.12. In a study with oleogel obtained using ethyl cellulose, olive oil, flaxseed oil, and fish oil, the color parameters of the emulsion were determined as CIE L* 25.9±0.1, CIE a* –0.1±0.1, and CIE b* 2.7±0.1 (Gómez-Estaca et al., 2019). In relation to textural attributes, the analysis revealed hardness and chewiness values of 0.21 N and 0.05 N, respectively. A research with chitosan observed chewiness values ranging from 2.68 to 7.28 N (Farooq et al., 2023). The polydispersity index (PdI) of the oleogel is 0.725. A PdI between 0−1 indicates a homogeneous and more stable system, while PdI>1 indicates high multiple distribution and instability (Tirgarian et al., 2023), suggesting that the oleogel has a homogeneous structure. Oleogel syneresis, observed with the separation of liquid from the gel, leads to an unstable formulation (Huri et al., 2013). In this study, the syneresis value of the oleogel was determined as 0.19%. The oleogel sample exhibited high thermal stability, with no phase separation observed in the oleogel structure at a temperature of 70°C for 1 h. The microscopic image of the oleogel is provided in Fig. 2. The distribution of oil globules within the water phase of the observed emulsion can be seen. In the image, the distinctive three-dimensional network structure of the oleogel is evident.
The proximate analyses, energy, and pH values of MEs are given in Table 3. The utilization of oleogel has been found to have an impact on the proximate composition and energy value of MEs. The highest moisture content was observed in the OG75 (63.65%) and OG50 (64.06%) groups, while the lowest moisture values were found in the C (61.92%) and OG100 (62.05%) (p<0.05). The addition of pre-emulsion, along with the inclusion of additional water in the formulation, is believed to contribute to the rise in moisture levels. Additionally, due to the higher TEF from the structure in OG100, lower moisture values were observed in this group. In a study, the moisture content of beef burgers increased with the utilization of olive oil oleogel-based emulsion (Özer and Çelegen, 2021). The lipid content of MEs varied between 9.82% (OG50) and 12.52% (OG100). There were no statistically significant differences between the lipid values of the groups in which beef fat was replaced with oleogels at 75% and 100% ratios and the control group (p>0.05). This is thought to happen because of the additional pre-emulsions, which constitute almost half of the mass in the lipid phase. Consistent with our findings, replacing pork fat in Bologna sausages with oleogels derived from sunflower oils has produced lipid values that exhibit no significant differences between the control and the treatments containing oleogels (Ferro et al., 2021). The protein content of the treatments varied between 15.26% (C) and 18.63% (OG75). The protein content of the MEs increased with the addition of oleogel regardless of the utilization ratio (p<0.05). The findings suggest that there is potential for augmenting the overall protein content through the application of chitosan-based oleogels. In a similar way, an increase in protein values has been observed in hamburgers where chitosan is used as a substitute for pork fat (Hautrive et al., 2019). There were no significant differences observed in the ash content among the MEs (p>0.05). Similar to our result, the ash contents of semi-smoked sausages were not affected by the addition of oleogels structure with beeswax (Igenbayev et al., 2023). The energy value of MEs varied between 183.50% (OG50) and 207.22% (C). The energy content of MEs was significantly affected by the addition of oleogel, and the highest value was detected in the control (p<0.05). This finding can be explained by the production of the control group using 100% beef fat. Reduced-fat beef burgers were produced using olive oil-based oleogel, and it was reported that there was a significant reduction in the total energy content (35%) in reformulated treatments (Özer and Çelegen, 2021).
The pH values of the emulsion samples ranged from 6.17 (OG50) to 6.22 (OG75). There was no statistically difference (p>0.05) observed between the pH values of the control group and the group where beef fat was replaced by 50% oleogel. Besides that, an increase in pH values was observed when beef fat was replaced by 75% and 100% oleogel (p<0.05). This can be attributed to the higher pH value of the oleogel (6.25).
The pH levels play a crucial role in influencing the quality characteristics (hardness, color, water holding capacity, etc.) of meat products (Young et al., 2004). Replacing beef fat with oleogel has been found significant on the pH values of MEs (Fig. 3).
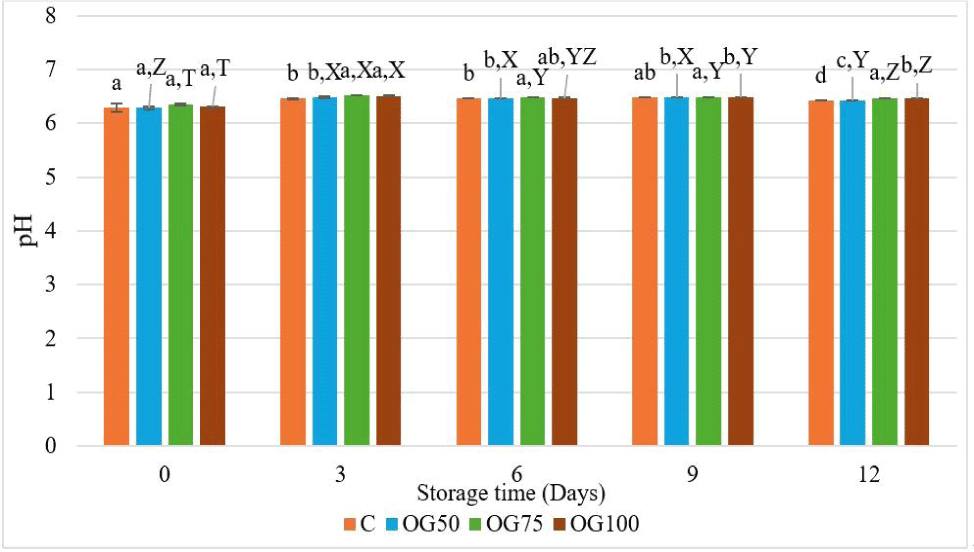
On the first day of storage, there was no significant difference observed among the pH values of the samples (p>0.05). However, on the 3rd day of storage, an increase in pH values was observed in the groups where beef fat was replaced by 75% or 100% oleogel (p<0.05). Starting from the 6th day, the highest pH value was found to be associated with OG75 (p<0.05). On the last day of storage (day 12), while the lowest pH value was observed in group C, the highest pH value was again observed in OG75. This can be attributed to the higher pH value of the oleogel (6.25). The control group did not show a significant difference during the storage period. Nevertheless, within the reformulated treatments, there was an initial increase in pH values throughout the storage period, followed by a subsequent decrease observed on the last day of storage (p<0.05). In some studies where oleogel was utilized as a substitute for animal fat, it was determined that there was no statistical difference among the pH values of the treatments (Igenbayev et al., 2023; Özer and Çelegen, 2021; Tarté et al., 2020).
Emulsion stability can be defined as the capacity of an emulsion to withstand alterations or changes over time (McClements and Jafari, 2018). A stable emulsion maintains fluid integrity within the system and displays a uniform structure under ideal conditions. The emulsion stability results from ME are presented in Table 4 as TEF% and expressible fat (EFAT%). The values of TEF ranged from 8.10% (C) to 31.38% (OG75). While the lowest TEF value was observed in the C, the highest values have been found in the OG75 and OG100 (p<0.05). Additionally, the substitution of oleogel as a beef fat replacer at a level of 75% and 100% did not show any significant difference among the groups (p>0.05).
Treatments1) | TEF (%) | EFAT (%) | JFS (%) |
---|---|---|---|
C | 8.10±0.68c | 14.24±1.07c | 13.87±0.87c |
OG50 | 11.34±0.74b | 15.69±0.72c | 14.45±0.42c |
OG75 | 31.38±0.87a | 22.57±1.26a | 24.75±0.86b |
OG100 | 31.21±0.90a | 19.50±0.40b | 30.54±0.70a |
The EFAT values of MEs were 14.24% (C) to 22.57% (OG75). When beef fat was substituted with oleogel at a level of 50%, the EFAT values of the treatments did not differ significantly from the control group (p>0.05). However, in groups where oleogel was used at 75% and 100%, an increase in these values was observed (p<0.05). Similarly, pork batters formulated with pork fat showed the highest water loss and fat loss (Shao et al., 2020). In another study, it has been reported that the use of oleogel as a fat substitute in meat products resulted in a decrease in TEF and EFAT values, leading to an improvement in emulsion stability (da Silva et al., 2019; Ferro et al., 2021; Özer and Çelegen, 2021). This situation varies depending on the formulation of the used oleogels.
The separation of JFS, indicating the total released liquid from emulsions at a specific temperature, serves as a significant indicator of emulsion stability (Serdaroğlu et al., 2016). In the model system MEs, the quantities of separated gel and fat, which are indicators of the stability of the emulsion dough following specific heat treatment, are presented in Table 4. There was no significant difference observed in the JFS values between the group in which beef fat was replaced with oleogel at a 50% ratio and the control group (p>0.05). On the other hand, the utilization of oleogel as a fat replacer at 75% and 100% ratios increased JFS values. This observation is consistent with the measurements of EFAT values conducted in the assessment of emulsion stability (Table 4). Consistent with our results, previous findings suggest that replacing animal fat may increase JFS levels (Nacak, 2020; Uzlaşır et al., 2020). Factors such as filler and binder type and quantity, production methods, raw material protein content, and pre-emulsion fat properties are thought to contribute to this effect.
In response to the increasing desire for healthier dietary choices, a notable strategy involves reducing fat content and concurrently adjusting the fatty acid composition in meat products. Table 5 presents the fatty acid composition of MEs, categorizing them according to nutritional ratios. Unsurprisingly, the replacement of beef fat with oleogel led to substantial differences in the fatty acid profiles of the samples, as evidenced by statistically significant variations (p<0.05). The addition of oleogel decreased the level of saturated fatty acids (SFA), from 58.07% (C) to 52.10% (OG100) (p<0.05). The possible explanation for this decrement is attributed to the oleogel’s fatty acid profile, as pomegranate seed oil is rich in punicic, linoleic, and oleic acids, as mentioned in the materials section. As the proportion of oleogel in the formulation increased, a rise in the Punicic-Linolenic acid values of the samples was observed (p<0.05), attributed to the high punicic acid content in pomegranate seed oil. The PUFA content of the samples increased with the higher oleogel ratio in the formulation, with the highest value observed in the OG100 (p<0.05). The fatty acid composition findings of our study are in line with studies conducted with different oleogels in formulated meat products (Ferrer-González et al., 2019; Ferro et al., 2021; Gómez-Estaca et al., 2019; Oh et al., 2019). Additionally, following European regulations (European Parliament, 2006), OG100 treatments may be classified as emulsified meat products characterized by “high unsaturated fat.” Additionally, they qualify for the nutritional claim of “high n-3 fatty acids” by containing over 0.6 g (0.72) of C18H30O2 per 100 g of the product. Changing the fatty acid component of products and lowering cholesterol is one of the main goals of the use of vegetable oils through emulsion in the meat industry. The cholesterol levels of MEs are presented in Table 5. According to the data, the lowest value (68.56 mg/100 g) was associated with the OG100 group, while the highest value (83.88 mg/100 g) was determined to belong to the control group (p<0.05). Replacing beef fat with oleogel containing pomegranate seed oil and chitosan resulted in a significant reduction in cholesterol content. There was no statistical difference in cholesterol levels between the OG50 and OG75 groups (p>0.05). In a study using oleogels derived from sunflower oil instead of pork back fat in the formulation of Bologna-type sausages, the cholesterol content in the control treatment, which was 44.3%, was found to be 41.2% in the group formulated with 100% oleogel (da Silva et al., 2019). In a study, where the animal fat in sweet sausage (Goon Chiang) was replaced with rice bran wax and rice bran oil oleogel at 25%, 50%, and 75% ratios, substituting 50% of the oleogel was reported to reduce total saturated fat and cholesterol content (Issara, 2022).
The incorporation of oleogel resulted in distinct texture profiles for the MEs (p<0.05) (Table 6). Hardness, springiness, cohesiveness, gumminess, and chewiness values were between 41.91–68.71 N, 0.10–0.15 mm, 0.12–0.18, 6.23–9.46 N, and 0.59–1.43 N.mm, respectively. The hardness values of reformulated samples were lower than the control (p<0.05). The reason for this is that the added oleogel is softer than beef fat, and the water and liquid oil in its composition can reduce hardness (Table 2). Consistent with our results, a higher hardness value was observed in the control on the production day in oil-reduced beef burgers prepared with an olive oil oleogel-based emulsion (Özer and Çelegen, 2021). The springiness values of treatments OG50 and OG75 exhibited higher values compared to C and OG100 (p<0.05). Parallel to our findings, springiness values of cooked meat batters increased with the addition of soybean oil oleogel as a fat replacer (Ferrer-González et al., 2019). In MEs, while the highest gumminess value was observed in OG50 (9.46 N), the lowest value was found in OG100 (6.23 N) (p<0.05). Besides that, no significant difference was observed between groups C and OG75 (p>0.05). Similarly, with the gumminess values, the OG50 had the highest chewiness value (1.43 N.mm), while the OG100 had the lowest value (0.59 N.mm). In a study, where linseed oil oleogel was used to replace pork back fat in Frankfurter sausages, it was reported that adhesiveness, gumminess, and chewiness values significantly increased by 50% in the group with oleogel substitution compared to the control (Franco et al., 2019). Researchers believe that adding oleogels to ME formulations is a better strategy than directly adding liquid oil, as it results in firmer products due to the small fat globules in the meat batter (Alejandre et al., 2019; Ferro et al., 2021).
The microstructure of MEs was evaluated using a SEM at a magnification of 10,000×. Fig. 4 displays micrographs of the MEs obtained from SEM. Formun ÜstüIt has been observed that treatments containing oleogel exhibited a smoother microstructure compared to the control. It is believed that the retention of pomegranate seed oil within the oleogel structure contributes to a uniform distribution within the ME matrix and enhances a compact structure. In a manner similar to our results, the utilization of glyceryl monostearate-based oleogels as a substitute for pork fat in Bologna sausage samples has been reported to result in a more compact appearance as the proportions of usage in the formulation increase (Ferro et al., 2021). In another study, it was reported that pork batters containing organogel with different oils (sunflower seed oil, peanut oil, corn oil, flaxseed oil) were more compact, while samples containing only pork fat exhibited more cracks and voids (Shao et al., 2020). Therefore, it can be stated that the addition of oleogel results in a more compact and continuous microstructure when compared to the control treatments.
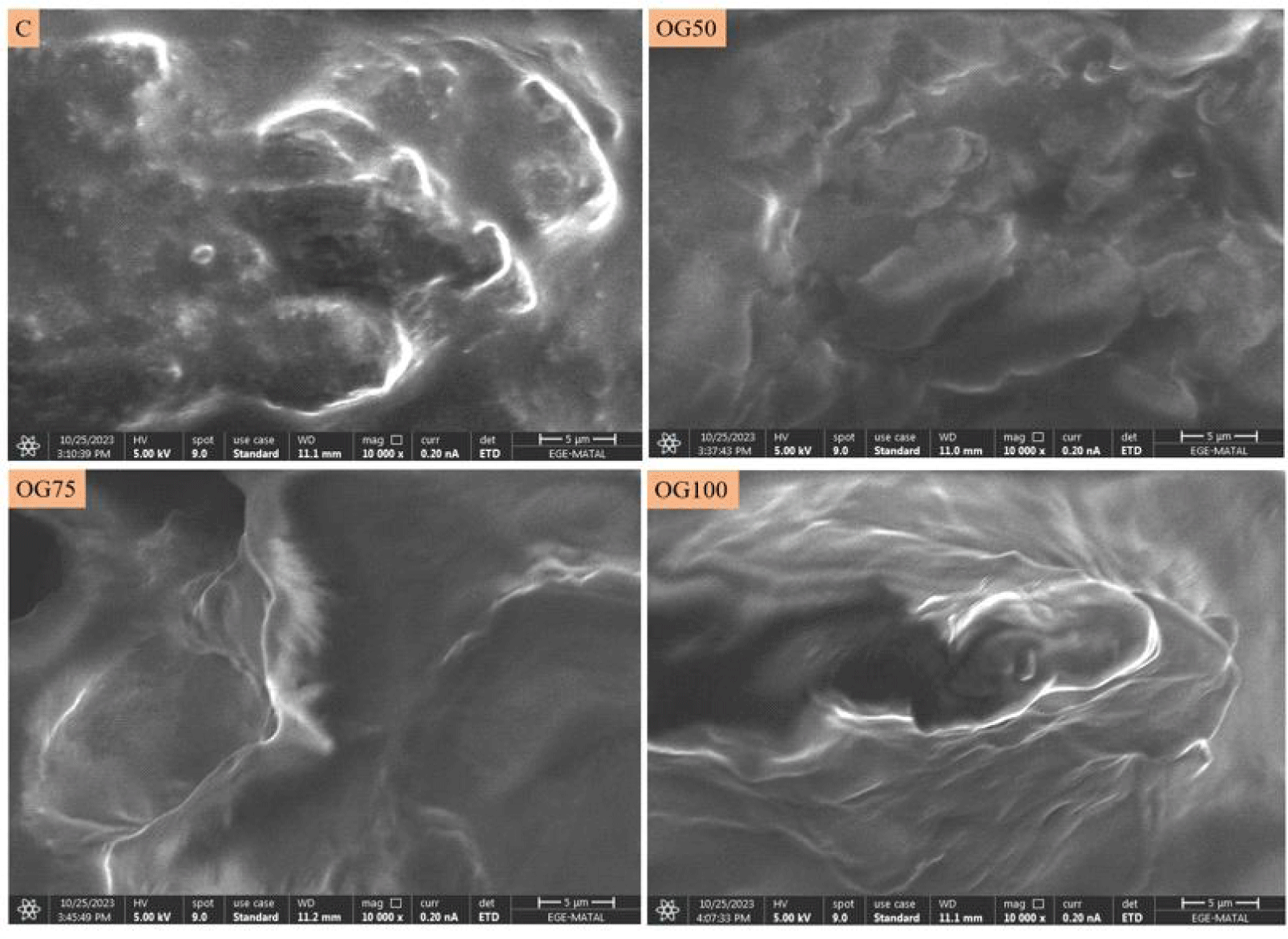
The main factor that affects consumers’ decisions to buy meat and meat products is their color. Color serves as an indicator of the product’s healthiness and freshness (Salueña et al., 2019). The color parameters of MEs measured during storage are presented in Table 7. The findings showed that the use of oleogel as a fat replacer significantly affected the color attributes (p<0.05). It has been determined that the CIE L*, CIE a*, and CIE b* values of MEs during storage ranged between 49.34–58.00, 12.59–15.89, and 7.52–9.45, respectively. The use of oleogel has significantly increased the CIE L* values of the treatments, and the highest CIE L* value was observed in OG100 during the 12-day storage period (p<0.05). Additionally, at the end of the storage, all treatments had higher CIE L* values compared to the first day of the storage. Similarly, in Bologna-type sausages where high oleic acid content oleogels were used as a fat replacer, it has been observed that treatments containing oleogel exhibited higher CIE L* values compared to the control group (da Silva et al., 2019). The utilization of oleogel in MEs leads to an elevation in product lightness due to the distinct distribution and light reflection characteristics of smaller fat globules in comparison to larger ones (Youssef and Barbut, 2009). Consequently, the average fat particle size decreases, resulting in an increase in CIE L* values.
On days 0 and 3, the CIE a* values of MEs decreased with the addition of oleogel to the formulation (p<0.05), while no significant differences were observed on days 6 and 12 (p>0.05). The reduction observed in the CIE a* values with the addition of oleogel can be attributed to the low CIE a* value (–2.93) of the oleogel (Table 2). In line with our results, in the case of Frankfurter-type cooked sausages, the use of chia mucilage-egg white-based oleogels at 0%, 25%, 50%, and 75% ratios led to a decrease in CIE a* values, attributed to an increase in oleogel content (Pérez-Álvarez et al., 2020). During the storage, fluctuations were observed in CIE a* values for all treatments, with an increase in the CIE a* values of C and OG100 on the last day of storage, while a decrease was detected in the CIE a* value of the OG75 (p<0.05). No significant difference was observed in OG75 treatment between the initial and final days (p>0.05).
The addition of oleogel to the formulation has increased the CIE b* values, regardless of the utilization ratio. Throughout storage, the lowest CIE b* value was observed in C (p<0.05). Researchers reported an increase in CIE b* values with the addition of oleogel samples in Bologna-type sausages (de Oliveira Faria et al., 2015; Gómez-Estaca et al., 2019). During the storage period, there was no statistical differences were observed in CIE b* values for the C and OG75 groups (p>0.05), whereas in the OG50 and OG100 treatments, CIE b* values initially decreased, then increased (p<0.05). At the end of the storage, the CIE b* value of OG50 decreased while an increment was observed in OG100 (p<0.05). The increase in the CIE b* value of OG100 during storage may be related to increased lipid oxidation (Shan et al., 2009). Franco et al. (2019) reported that the addition of oleogel increased the CIE b* value, attributing this increase to the additives used in oleogel production. They also noted that the addition of oleogel to Frankfurter sausages resulted in an increase in the CIE b* value from 16.61 to 18.85.
Oxidation is the main cause of deterioration in both liquid and solid fats, revealing harmful substances and diminishing the food’s shelf life, sensory appeal, and nutritional value. Lipid oxidation in meat products has been assessed using TBAR analysis, a method that detects malondialdehydes as secondary oxidation products (Poyato et al., 2015). The TBAR values of MEs during storage are presented in Fig. 5. The utilization of oleogel had a significant effect on lipid oxidation, the highest TBAR value was recorded in control throughout the storage. Moreover, the lowest value was observed in OG100 on the 0th and 3rd days of the storage (p<0.05). Researchers reported that oleogelation resulted in a reduced rate of oxidation in both oleogels and oleogel emulsions (da Silva et al., 2019; Pan et al., 2021). On the 6th day, reformulated treatments exhibited lower TBAR values compared to the control group, regardless of the utilization ratio (p<0.05). The treatment containing 100% oleogel showed the lowest TBAR value, believed to be attributed to chitosan present in the oleogel. Studies conducted on pork and spiced beef have revealed that chitosan inhibits lipid oxidation (Koç and Özkan, 2011). In reduced fat beef burgers prepared with olive oil oleogel-based emulsion, the results of 7-day storage indicated a gradual increase in TBAR value for all beef burger treatments. Similarly, control treatments showed higher TBAR values compared to burgers containing olive oil oleogel throughout the storage period (Özer and Çelegen, 2021). Contrary to our findings, higher TBAR values were observed in Frankfurt-type sausages where pork back fat is replaced with chia-mucilage egg white-based oleogels at rates of 50% and 75% (Pérez-Álvarez et al., 2020).
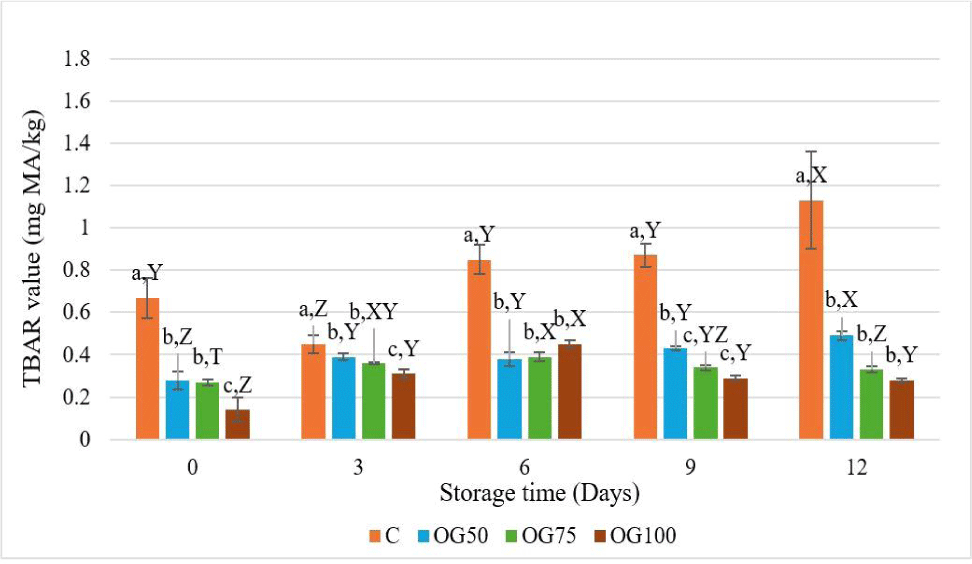
The rise in protein carbonyls indicates the susceptibility of muscle proteins to oxidative processes leading to an increase in carbonyl content. Therefore, total protein carbonyl content is used as a marker of protein oxidation (Ergezer and Serdaroğlu, 2018). The effects of oleogel addition and storage on the carbonyl levels of MEs are presented in Fig. 6a. At the beginning of storage, it was determined that the carbonyl levels of MEs ranged from 0.42 (OG75) to 2.22 nmol/mg protein (C). The highest carbonyl level was obtained in C treatment (p<0.05), on the other hand, there was no statistical difference between the OG50, OG75, and OG100 groups (p>0.05). Except for the 0th and 9th days, the lowest carbonyl content was detected in OG100 (p<0.05). Chitosan, a versatile biopolymer, has been reported to have antioxidant and antimicrobial activities (Morachis-Valdez et al., 2017). A study has demonstrated that chitosan has the potential to influence the sulfhydryl and carbonyl content of proteins. Specifically, the application of chitosan grafted chlorogenic acid has been found to inhibit the formation of free amino acid and carbonyl groups maintaining a higher sulfhydryl content and thereby retarding protein oxidation (Yang et al., 2022). It was found that the storage period led to an increase in carbonyl content in the treatments. Throughout the storage period, the highest average carbonyl content was observed in the C treatments (p<0.05). During the 12-day storage, carbonyl levels varied between 0.42 (OG75) and 14.70 nmol/mg protein (OG50). The lowest value was observed in OG75, while the highest carbonyl value was observed in OG50 (p<0.05). In contrast to our findings, Agregán et al. (2019) investigated the impact of Fucus vesiculosus extracts, serving as natural antioxidants in pork patties formulated with oleogels, over an 18-day storage period. They observed a gradual and sustained rise in carbonyl content.
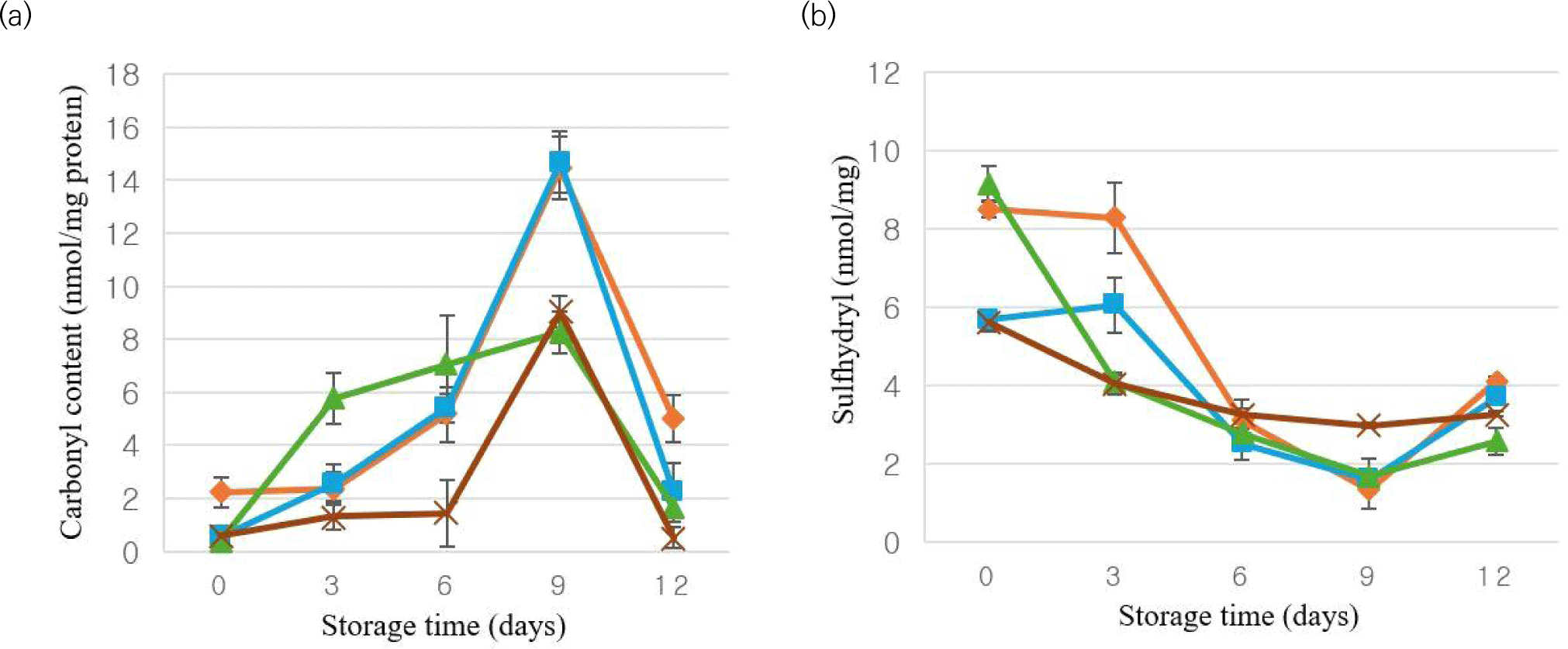
Sulfhydryl groups of cysteine amino acids are extremely sensitive to oxidative changes. Various oxidized compounds, including sulfenic acid and sulfinic acid, are formed when sulfhydryl protein groups in meat and meat products enter into complex reactions (Domínguez et al., 2021). As a result, measuring losses in sulfhydryl groups is an important analysis used to determine the degree of protein oxidation in meat products (Rather et al., 2016). Sulfhydryl content during the storage period of MEs is given in Fig. 6b. The use of oleogel has been found to affect the sulfhydryl levels of the MEs. On the first day of storage, sulfhydryl levels varied between 5.62 (OG100) and 9.15 (OG75) nmol/mg protein. The highest sulfhydryl concentration was noted in control treatment on days 3 and 12 of storage, whereas on days 6 and 9, it was observed in OG100 (p<0.05). Overall, a decrease in sulfhydryl levels of all treatments was observed during the storage period, attributed to a general increase in protein oxidation. Previous study has indicated that the antioxidants effective against lipid oxidation may not always be effective against protein oxidation (Nacak et al., 2021).
Conclusion
The results of this study have demonstrated the feasibility of using beef fat replacement in oleogels prepared with chitosan and pomegranate seed oil at substitution rates not exceeding 50%. The replacement of beef fat with oleogel at a ratio exceeding 50% has led to a decrease in the emulsion stability of the MEs. On the other hand, the incorporation of oleogel resulted in a decrease in total fat, SFA, and cholesterol content, accompanied by an increase in both mono and PUFAs. Despite a higher level of protein oxidation observed in the reformulated samples, the reformulation process appeared to counterbalance the changes in lipid oxidation. Nevertheless, incorporating oleogel into the formulation presents challenges, especially concerning color and enhanced stability. Future studies should focus on examining the effects of incorporating pomegranate seed oil in oleogel formulations with different components on the sensory and technological quality attributes of meat products.