서 론
나노 에멀젼 전달체(nano emulsion delivery system)는 1–200 nm 크기를 지닌 아주 작은 크기의 전달체로 정의할 수 있으며, 유식품 제조 공정 및 저장, 소화 기간 동안 생리 활성 화합물(bioactive compound)을 보호하고 이용성을 극대화할 수 있다(Fathi et al., 2012; Ron et al., 2010). 소수성(hydrophobic) 생리 활성 화합물은 건강 기능성 향상에 중요한 역할을 하지만, 식품 가공 및 저장조건, 위장 소화 과정 중 낮은 용해도 및 물리화학적 안정성 저하로 인해 생체 이용률(bioavailability)이 낮아 유식품 적용 시 한계가 있다(Bryant and McClements, 1998). 이러한 소수성 생리 활성 화합물의 유식품 적용 시 발생 가능한 문제점들을 해결하기 위해 이용되는 나노 에멀젼 전달체는 매우 작은 입자크기로 인해 표면적이 넓기 때문에 소화관 내 용해도가 증가하고, 위장 체류 시간을 연장하여 생리 활성 화합물의 생체 이용률을 향상시킬 수 있으며, 식품 가공 및 저장조건 하에서의 생리 활성 화합물의 안정성을 향상시킬 수 있다(Banerjee et al., 2016; Han et al., 2008; Kohane, 2007).
유단백질(milk protein)은 대략 80% 케이신(casein)과 20% 유청 단백질(whey protein)으로 구성되어 있다. 그중 유청 단백질은 α-lactalbumin, β-lactoglobulin, bovine serum albumin, lactoferrin, immunoglobulin으로 구성되어 있으며, 필수아미노산을 다량 함유하고 있어 영양학적 가치가 뛰어나다(Livney, 2010; Sun and Gunasekaran, 2009). 유청 단백질은 낮은 독성, 생체 적합성 및 생분해성이며, 겔화(gelation) 능력을 지니고 있다. 또한 낮은 수용해도로 인해 유식품 적용에 문제점을 지니고 있는 docosahexaenoic acid(DHA), eicosapentaenoic acid(EPA) 등과 같은 소수성 생리 활성 화합물에 대한 우수한 결합능력으로 인해 나노 전달체 제조 시 surface coating material로 이용되어 왔다(Adjonu et al., 2014; Ha et al., 2019; Shafaei et al., 2017). 그러나 유청 단백질은 제조공정 요인(예: 온도, pH)에서 구조적 특성 변화로 인한 유화 능력 저하로 나노 에멀젼 적용 시 문제점을 지니고 있다(Capanni et al., 2004; Li and Gu, 2011; Luo et al., 2011; Yi et al., 2006). 본 리뷰에서는 이러한 유청 단백질을 나노 에멀젼 전달체의 coating material로 이용 시 발생 가능한 문제점들을 최소화하기 위해 유청 단백질을 이용한 마이야르 반응(Maillard reaction)을 기반으로 생성되는 공액체(conjugate)를 이용하여 유청 단백질의 물리화학적 기능성(physicochemical functionality)을 향상시켜 궁극적으로 나노 전달체의 생리 활성 화합물 이용성을 증가시키는 최신 기술 트렌드에 대해 논하고자 한다.
마이야르 공액체
마이야르 반응은 식품을 저장하거나 가열하는 동안 단백질과 다당류 사이에서 발생하는 비효소적 갈변 반응(non-enzymatic browning)이며, 마이야르 반응의 초기 단계에서는 N-glycosylamines을 형성하는 아미노산/펩티드/단백질의 유리 아미노기와 환원당의 카르보닐기 사이의 축합(condensation)이 진행되면서 불안정한 N-glycosylamines은 Amadori rearrangement을 거쳐 ketosamine을 형성한다. 앞서 생성된 ketosamine는 reductone과 diacetyl, pyruvaldehyde 및 기타 단쇄 가수 분해 생성물들을 형성한다. 마지막 단계에서 reductone 및 가수분해 산물, Strecker 분해 산물은 aldol과 aldehyde-amine 축합 반응을 거쳐 궁극적으로 갈색 지소 고분자인 melanoidin을 생성한다(Hodge, 1953; Peng et al., 2011). 마이야르 반응 결과 생성된 melanoidin은 향상된 건강 기능성 특히 항산화, 항균 기능을 지니고 있다. 그러나 마이야르 반응의 최종 단계가 오래 지속되면 당뇨병 및 알츠하이머와 같은 질병 유발 요인이 될 수 있기 때문에 마이야르 반응을 고압(400 MPa)에서 수행하거나 pH를 8.0보다 낮게 설정하여 질병 유발 요인 형성을 억제할 수 있다(de Oliveira et al., 2016; Moreno et al., 2003; Silván et al., 2011).
마이야르 반응을 통해 생성된 마이야르 공액체(Maillard conjugate)는 단백질과 다당류의 공유결합으로 형성되며, 기존 단백질보다 우수한 용해도 및 유화 능력, 항산화 특성으로 인해 전달체의 coating material로 사용 시 기능적 특성(functional properties) 향상을 통해 다양한 긍정적인 효과를 기대할 수 있다(Nooshkam and Madadlou, 2016a; Nooshkam and Madadlou, 2016b; Vhangani and Van Wyk, 2013; Vhangani and Van Wyk, 2016). 마이야르 공액체의 여러 기능적 특성은 공액체 제조 공정 요인인 마이야르 반응 시간과 열처리 온도 및 pH, 원료, 단백질과 다당류의 초기 비율 등에 영향을 받는다(Table 1). Wang 등(2020)의 유청 단백질(whey protein isolate, WPI)과 이눌린 공액체의 연구에서 pH가 3.0에서 7.0으로 증가함에 따라 공액체의 유화 특성이 유의적으로 증가하였으며, 특히 유화 안정성 실험에서 pH가 증가함에 따라 큰 증가가 나타났다. 또한 마이야르 공액체 제조 시 마이야르 반응 시간이 2시간에서 6시간으로 증가함에 따라 항산화 특성인 ABTS [2,2'- azino-bis(3-ethylbenzothiazoline-6-sulfonic acid)] radical 소거 활성이 유의적으로 증가하였다.
Parameters | Functional property | Reference | |||||
---|---|---|---|---|---|---|---|
Protein (P) | Carbohydrate (C) | Ratio P:C | Temp (°C) | Time(h) | pH | ||
Whey protein isolate | Inulin | 1:1, 2:1, 4:1, 5:1, 6:1 | 70, 75, 80 | 12, 24, 48, 72 | 7.0 | Thermal stability emulsifying properties | He and Vardhanabhuti (2021) |
Gum acacia | 1:2 | 60 | 24, 72, 120, 168 | - | Thermal stability emulsifying properties | Chen et al. (2019) | |
Inulin | 1:1 | 70 | 2, 4, 6 | 9.0 | Emulsifying properties oxidative stability | Wang et al. (2020) | |
Flaxseed gum | 3:1 | 60 | 22, 48, 72 | 7.2–7.4 | Thermal stability emulsifying properties | Dong et al. (2020) | |
Whey protein | Inulin | 3:1, 2:1, 1:1, 1:2, 1:3 | 60 | 120 | 7.0 | Oxidative stability | Wang et al. (2019) |
마이야르 공액체의 기능적 특성
유청 단백질은 고온 열처리 공정[예: 일반적인 상업용 발효유 열처리(85°C/30분, 95°C/10분, 상업용 우유 멸균(138°C/4초)]에서 고온으로 인한 구조적 변화 및 침전으로 인해 유청 단백질의 기능적 손실을 초래할 수 있다(de Oliveira et al., 2016; Rinaldi et al., 2014; Wang et al., 2006). 유청 단백질과 환원당 간의 마이야르 반응 결과, 형성된 마이야르 공액체는 열처리 공정 시 유청 단백질 변성으로 인한 단백질 간의 intermolecular hydrophobic interaction을 감소시켜 열처리로 인한 유청 단백질의 구조적 변화를 억제할 수 있다(Sasahara et al., 2003). 소수성 부분의 결합을 방해하여 단백질의 구조를 보호하며 또한 마이야르 공액체 형성에 음이온성 다당류를 이용할 경우, 입체 장애(steric hindrance) 및 정전기적 반발(electrostatic repulsion)로 인해 결합된 단백질의 열 안정성을 증가시켜 열처리시 단백질의 응집을 방지할 수 있다(Liu et al., 2019). Jiménez-Castaño 등(2007)의 유청 단백질인 β-lactoglobulin(β- Lg)과 환원당인 dextran 마이야르 공액체를 이용한 열 안정성 연구에서 β-Lg만을 이용하였을 경우와 비교하여 β-Lg/dextran 마이야르 공액체의 pH 5.0 및 7.0에서 열 안정성이 유의적으로 더 높았다. 또한 분자량이 다른 dextran에 대한 β-Lg/dextran 마이야르 공액체의 열 안정성 연구에서는 dextran이 20 kDa인 β-Lg/dextran 마이야르 공액체의 열 안정성은 dextran이 10 kDa인 공액체보다 유의적으로 높았는데, 이러한 결과는 고분자 탄수화물의 입체 반발이 저분자 탄수화물의 입체 반발에 비해 높기 때문이다(O’Mahony et al., 2017).
마이야르 반응을 이용한 마이야르 공액체 형성은 단백질의 유화 특성(emulsifying properties)을 향상시키는 것으로 알려졌다(Feng et al., 2016). 마이야르 공액체의 단백질 부분은 O/W 에멀젼(oil in water emulsion)의 oil droplet 표면에 빠르게 흡착되고, 공액체의 환원당 부분은 입체 장애(steric hindrance) 및 정전기적 반발력(electrostatic repulsion)을 통해 oil droplet의 응집을 억제할 수 있어 결과적으로 유화 특성을 향상시킬 수 있다(Gumus et al., 2016). Wang 등(2020)의 native WPI 및 WPI-Inulin 마이야르 공액체를 이용한 유화 활성 지수(EAI; emulsifying activity index) 및 유화 안정성 지수(emulsion stability index, ESI) 실험에서 WPI-Inulin 마이야르 공액체의 유화 활성 지수는 native WPI의 유화 활성 지수보다 유의적으로 상당히 높았다. 이러한 유화 활성 증가는 WPI-Inulin 마이야르 공액체가 당화 반응을 통해 사슬 형태가 확장되고, 표면 구조가 느슨하게 되어 결과적으로 O/W 에멀젼의 기름-물 계면(oil-water interface)에서 흡착(adsorption)을 더 빨리 촉진했기 때문입니다(Mu et al., 2006; Xu and Yao, 2009). 또한 WPI-Inulin 마이야르 공액체의 유화 안정성 지수(ESI)는 pH 3.0–7.0 범위에서 native WPI보다 유의적으로 높았는데, 이러한 향상된 유화 안정성은 WPI-Inulin 마이야르 공액체가 native WPI와 비교하여 상대적으로 높은 정전기적 반발력을 지니고 있어 보다 안정적으로 에멀젼 droplet 표면에 흡착하고, 에멀젼 형성 동안 droplet 응집(flocculation) 속도를 느리게 하기 때문이다(Consoli et al., 2018; Yang et al., 2018). 이와 같이 유청 단백질 유래 마이야르 공액체의 우수한 유화 특성은 에멀젼 형태의 전달체에서 천연 유화제로의 역할을 할 수 있음을 의미한다.
마이야르 공액체의 항산화 특성은 유식품 저장 기간 동안 소수성 생리 활성 화합물(예: DHA, EPA 등)의 산화적 산패(oxidative rancidity) 관련 문제를 최소화할 수 있다(Nooshkama et al., 2020). 마이야르 반응을 이용하여 단백질과 환원당으로 제조된 마이야르 공액체의 항산화 특성 연구에서 마이야르 반응으로 생성되는 Amadori 유래 성분, reductone 및 melanoidin과 같은 마이야르 반응 생성물(Maillard reaction production: MRP)에서 우수한 항산화 효과가 보고되었으며, 마이야르 공액체의 항산화 활성은 분자량, pH, 온도, 반응시간 등을 포함한 많은 요인의 영향을 받는다(Franzke and Iwainsky, 1954; Nooshkam et al., 2019). 예를 들면 마이야르 반응 생성물인 melanoidin은 분자량에 따라 항산화 활성이 달라지는데, 상대적으로 긴 마이야르 반응시간에서 생성된 고분자 melanoidin은 저분자 melanoidin보다 항산화 활성이 높다고 보고되었다(Wang et al., 2011). 이러한 마이야르 반응 생성물의 항산화 특성은 자동산화(autoxidation) 과정 중 radical chain reaction을 종결하기 위한 hydroxyl pyrrole 그룹의 전자 전달활성(electron transfer activity) 기능, 금속이온 킬레이트화, H2O2 파괴 효과 및 활성 산소 포집 기능을 통해 향상된다고 알려져 있다(Nooshkam and Madadlou, 2016; Wang et al., 2013). 마이야르 공액체는 건강 기능성 불포화 지방산인 DHA 등을 함유한 기능성 유제품의 산화 반응을 억제하는 잠재적 항산화제로 사용 가능하다(Giroux et al., 2010; Nooshkam et al., 2020).
소화 과정 동안 생리 활성 화합물의 생체 이용률을 증진시키기 위해 위장 내 조건에서 생리 활성 화합물의 물리화학적 안정성 및 생체 접근성 향상, 분해 감소 및 흡수 개선 등이 필요하다(Chen et al., 2018). 단백질과 환원당 유래 마이야르 공액체를 이용하여 제조된 에멀젼 전달체는 천연 단백질에 의해 안정화된 에멀젼 전달체와 비교하여 위장 내 소화과정(예: pH 1.0–3.0 및 펩신에 의한 단백질 분해) 동안 생리 활성 화합물에 보다 높은 protection effect를 제공하는 것으로 알려졌다(Gumus et al., 2016; Lesmes and McClements, 2012; Xu et al., 2014; Zhong et al., 2019). 마이야르 공액체는 O/W 에멀젼 전달체의 oil droplet 주위에 두꺼운 계면안정층(interfacial layer)을 형성하고, 이렇게 형성된 계면안정층이 에멀젼 droplet과 펩신 간의 상호 작용을 억제하고, 결과적으로 위장 내 소화과정에서 생리 활성 화합물을 보호한다(Davidov-Pardo et al., 2015). 케이신-말토덱스트린(casein-maltodextrin) 마이야르 공액체를 이용한 전달체의 위장 소화 연구 결과, 말토덱스트린 공액체의 계면 안정층으로 인한 barrier effect로 인해 전달체 내 포집된 소수성 생리 활성 화합물이 pepsinolysis로부터 보호되었다(Markman and Livney, 2012). 또한 소화 과정 동안 생리 활성 화합물의 생체 이용률을 증진시키는 요인에는 마이야르 공액체의 barrier effect뿐만 아니라, 마이야르 공액체를 이용하여 제조된 에멀젼 전달체의 droplet size가 전달체에 의해 포집된 생리 활성 화합물의 생체 이용률에 영향을 미친다. Fig. 1은 WPI와 Inulin 마이야르 공액체를 이용하여 제조된 O/W 에멀젼 전달체를 투과전자현미경(transmission electron microscope, TEM)으로 관찰한 결과이며, droplet size가 대략 100 nm인 것을 확인할 수 있다. 나노미터 크기의 에멀젼 전달체는 증가된 표면적으로 인해 소장의 mucosal adhesion과 장내 상피세포와의 상호작용을 증진시킬 수 있으며(Banerjee et al., 2016; Yao et al., 2015). 그 결과 포집된 생리 활성 화합물의 생체 이용률을 증가시킬 것으로 예상된다(Acosta, 2009; Fathi et al., 2012; Ha et al., 2015).
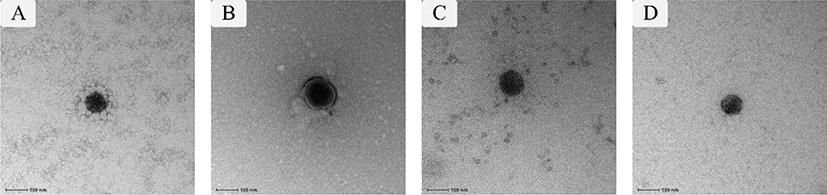
결 론
생리 활성 화합물은 건강 기능성 향상에 중요한 역할을 하지만, 식품 가공 및 저장조건, 위장 소화 과정 중 낮은 용해도 및 물리화학적 안정성 저하로 인해 생체 이용률(bioavailability)이 낮아 유식품 적용 시 한계가 있다. 유청 단백질과 환원당을 반응시킨 마이야르 공액체는 우수한 열 안정성, 유화 능력, 및 항산화 특성을 지니고 있어 나노 에멀젼 전달체의 coating material으로 이용 시 식품 가공 및 저장조건, 위장 소화 과정 중 소수성(hydrophobic) 생리 활성 화합물의 생체 이용률을 증진시킬 수 있는 효과적인 기능성 식품 소재이다.